It’s long been the dream of humanity to escape the bonds of Earth’s gravitational pull, paving the way for us to explore the vast reaches of space that lie beyond our world. Beginning in the 20th century, we started to achieve this dream by leveraging the power of rocket technology, where we’d burn fuel to provide a large and constant acceleration to a payload, eventually taking it above Earth’s atmosphere and either into orbit around our planet or — more ambitiously — to escape from our planet’s gravity entirely.
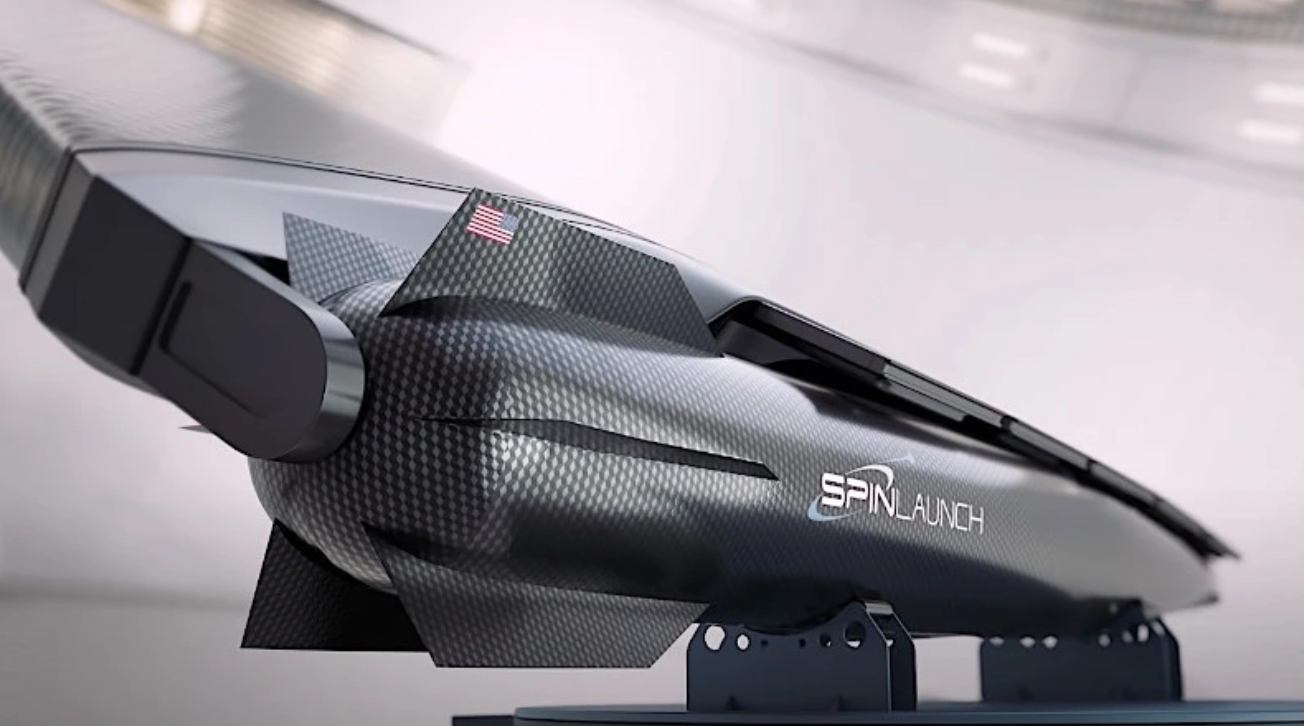
However, rocket launches, even when the launch vehicle is salvageable and reusable, are tremendously resource-intensive, expensive, and environmentally unfriendly. Since the mid-20th century, numerous alternative technologies have been proposed to send objects to space, but none have yet achieved that goal as of 2022. One company aims to change that in the coming few years: SpinLaunch. Ideally, they’ll build a full-scale version of their modestly-sized working prototype to spin objects up to speeds of 5,000 miles-per-hour (8,100 kph) and launch them upward, where a small booster will take them all the way into space. It’s an ambitious goal, but the laws of physics might be standing in the way. Here’s why.
A few ideas have been offered as alternatives to rocket launches over time.
- A railgun, for instance, would electromagnetically accelerate a projectile along a track until the projectile reached the end, where it could potentially reach space with a large enough exit velocity.
- A space elevator, alternatively, would lift an object intended for orbit all the way up above Earth’s atmosphere, relying on solid-enough infrastructure to carry a payload without a launch vehicle.
- Or a ballistic solution, where an object is simply fired at high velocity upward through the atmosphere, could take an object most of the way or even completely into space.
That last option, through the 1950s and 1960s, gave rise to Project HARP: the High Altitude Research Project, which once sent a gun-fired projectile up to the highest altitude ever achieved by that means, to 180 kilometers (110 miles) above the surface of the Earth. However, a combination of factors — including the trauma experienced by the payload during its initial firing — prevented a late-stage rocket booster from functioning as part of the payload, prohibiting it from reaching orbit or from escaping Earth’s gravity.
It’s very likely that Project HARP served as the inspiration for what SpinLaunch is attempting to do today.
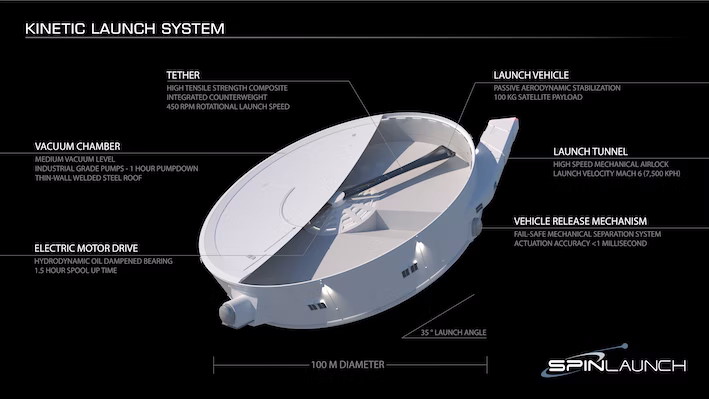
The idea of SpinLaunch is both devilishly simple and incredibly complex. Instead of using a gun-based launch like Project HARP did, SpinLaunch will build a large circular accelerator, kind of like a centrifuge. On one end, a payload inside an aerodynamic craft is prepared; on the other end, a counterweight balances it out. The air inside is evacuated, creating a vacuum. And then, the spin-up begins. With each revolution inside the SpinLaunch mechanism, the payload and counterweight speed up, increasing their angular velocity over and over.
Once a critical speed is reached, the payload detaches from the rest of the apparatus and is launched straight upward, where it penetrates a thin but airtight sheath, entering Earth’s atmosphere. The goal isn’t to go all the way up into space, but rather “only” to extremely high altitudes, not only above Earth’s troposphere and into the stratosphere, but even above the stratosphere and all the way into the mesosphere. Only then will a booster rocket kick in and take the payload the rest of the way into space, saving an enormous amount of fuel costs and launch costs overall. Ideally, SpinLaunch will be capable of launching many payloads each day, at a fraction of the cost of even reusable rocket launches.
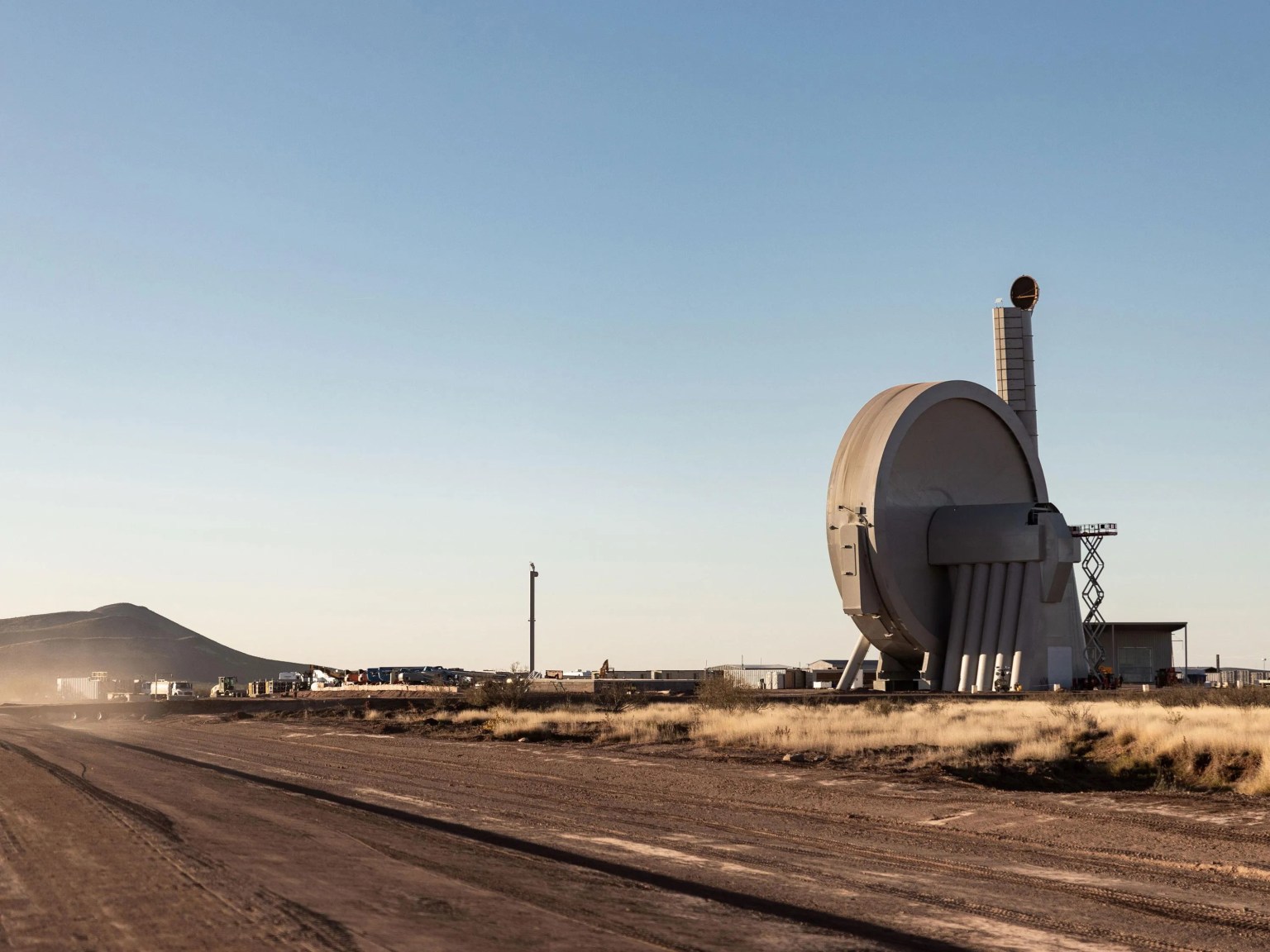
So far, SpinLaunch has built two prototypes, the largest of which is a third the diameter of the desired final version. Already, this prototype has successfully launched test payloads:
- that have successfully detached at the right moment,
- that have successfully pierced a mylar membrane maintaining the vacuum,
- at exit speeds of approximately 1,000 mph (1600 kph),
- where the payload has then reached heights of ~30,000 feet, or nearly 10 kilometers.
This is remarkable and impressive, but not necessarily impressive enough. In order to successfully enter low-Earth orbit, a spacecraft needs to reach altitudes of around 300 kilometers (186 miles) with orbital speeds of 25,000 kph (16,000 mph), which implies much greater speeds and heights than SpinLaunch has been able to reach. To get there, the plan is to have the full-scale SpinLaunch system achieve exit speeds of 5,000 mph (8,100 kph), and to then have a working late-stage rocket activate to take the payload the rest of the way into orbit once it reaches a height of ~60 kilometers.
Will SpinLaunch be a feasible concept when it’s scaled up to its desired design? That all depends on whether the following physical problems can be overcome.
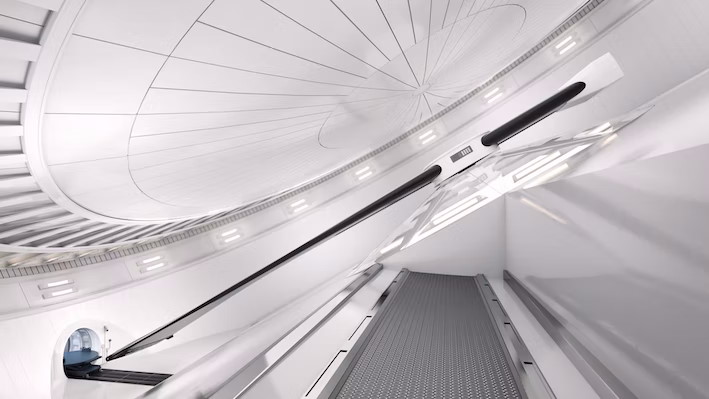
Problem #1: Can the payload survive spin-up?
This is not a trivial problem. Whenever you accelerate an object to move in a circle, it experiences not only the “spin-up” force that causes its angular speed to increase, but also a centripetal force — a force toward the center of the circle — that prevents the object from either crashing into the side of the accelerator or from flying off in a straight line prematurely. That centripetal force is dependent on three factors:
- the mass of the payload,
- the speed of the object,
- and the size (radius) of the circle.
At its full-scale size and with a desired exit speed of 5,000 mph (8,100 kph), that translates into a peak centripetal acceleration, just before the payload is launched, of somewhere between 50,000 and 100,000 gs, where one g is the acceleration due to gravity at Earth’s surface. The payload must ramp up to this peak acceleration over long periods of time — something like ~30 minutes — and survive it with all systems intact, including the on-board rocket system, in order to reach orbit. This represents a peak acceleration that’s eight times what the current prototype experiences.
Such conditions have never been met to date; this is a tremendous obstacle to be overcome.
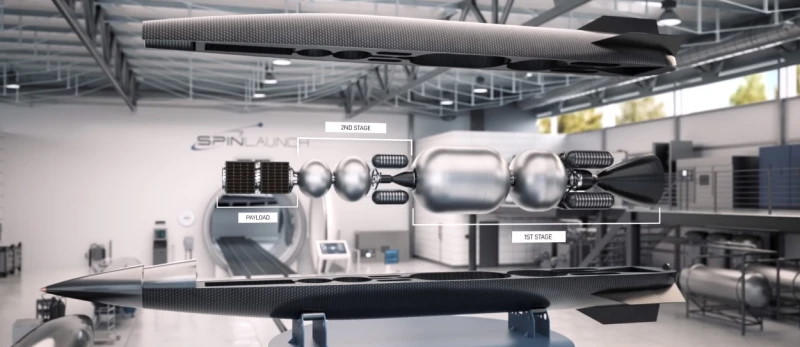
Problem #2: Traditional liquid-based rocket fuel cannot be used.
It’s always preferable to build upon already-existing technologies than it is to have to invent something entirely new, and yet the latter is very much what’s in store for a SpinLaunch payload. The reason is simple: if you have a liquid-based fuel on board, you need a plumbing system to contain and control it; this is exactly the type of system that will not survive the spin-up accelerations that SpinLaunch requires.
This means that solid rocket fuel will need to be used instead: something with the hardness and durability of something like formica. In principle, this can be done, but it represents a substantial obstacle toward reaching space.
While solid-propellant rockets offer a number of advantages over liquid-propellant ones, those advantages include stability, durability, and reliability. Unfortunately, however, they have lowered efficiencies and are less controllable than liquid propellant alternatives, which is why solid-fuel rockets are primarily used in military armaments but liquid-fuel rockets are typically used for spaceflight. Even if this difficulty can be overcome, the limitations of solid-fuel applications will inherently limit the mass of the payloads that can be delivered with SpinLaunch.
Problem #3: Piercing the mylar sheet preserving SpinLaunch’s vacuum could destroy the payload.
Do you remember the unfortunate and tragic Space Shuttle Columbia disaster? When Columbia attempted atmospheric re-entry, the spacecraft catastrophically broke apart, killing all astronauts on board. The reason the shuttle disintegrated in the atmosphere, however, was simply a small, lightweight piece of foam insulation that struck a portion of the craft at very high speeds. That’s a key concept in physics: the amount of kinetic energy that something possesses — and hence, the amount of damage it can cause in a collision — is proportional to its mass, but proportional to its speed squared.
With an exit velocity of 5,000 miles-per-hour as opposed to the 1,000 miles-per-hour of the current prototype, that means:
- the launch vehicle will strike the mylar sheet with 25 times the kinetic energy of current tests,
- the mylar sheet will impart 25 times the amount of energy as current tests to the payload,
- and the transition from the payload traveling through vacuum to traveling through Earth’s atmosphere means “hitting a wall” of atmosphere that the payload will strike with 25 times the amount of force that the current prototype experiences.
Can the launch vehicle/payload survive this set of conditions and remain fully operational and undamaged? It’s possible, but it’s never been done before. Again, this is an unprecedented obstacle that must be overcome.
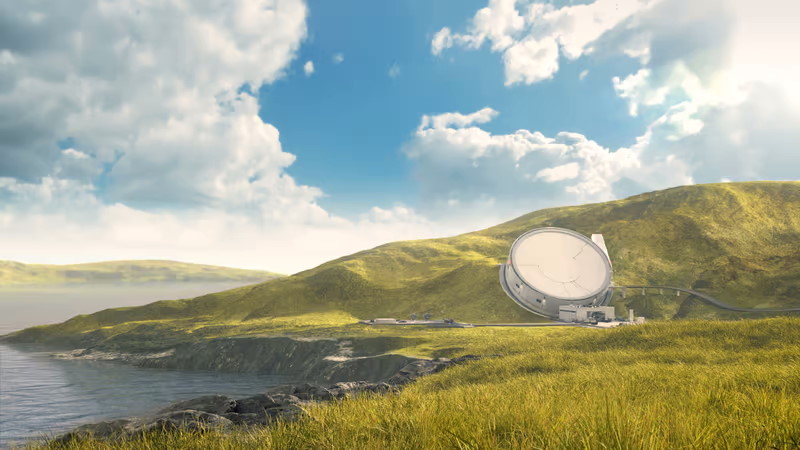
Problem #4: The atmospheric drag force experienced by the payload will be tremendous.
If you hold your hand out of a car window when you’re traveling at 100 kph (62 mph), how much of a drag force will it experience relative to traveling at half that speed: 50 kph (31 mph)? The answer isn’t twice as much force, as one might expect, but rather four times as much force. The drag force you experience is proportional to the cross-sectional area of the object (your hand, in this case) but also to the velocity you’re moving at squared.
Normally, rockets start out moving slowly near Earth’s surface — where the atmosphere is thickest — and pick up speed as they continue accelerating up through the atmosphere. The highest speeds are achieved at the highest altitudes: where the air is thinnest.
Not so with SpinLaunch; in fact, the reverse is true. The payload will be moving at its fastest where the atmosphere is the thickest, which maximizes speed and energy losses due to drag. This will also heat up the payload substantially, and in ways that no payload that’s ever made it to space before has experienced. The biggest problem with Project HARP, back when it was being run, is that there was no payload that could be launched that would be capable, at its high altitude, of taking it the rest of the way to space. Can SpinLaunch overcome that problem? It remains to be demonstrated.
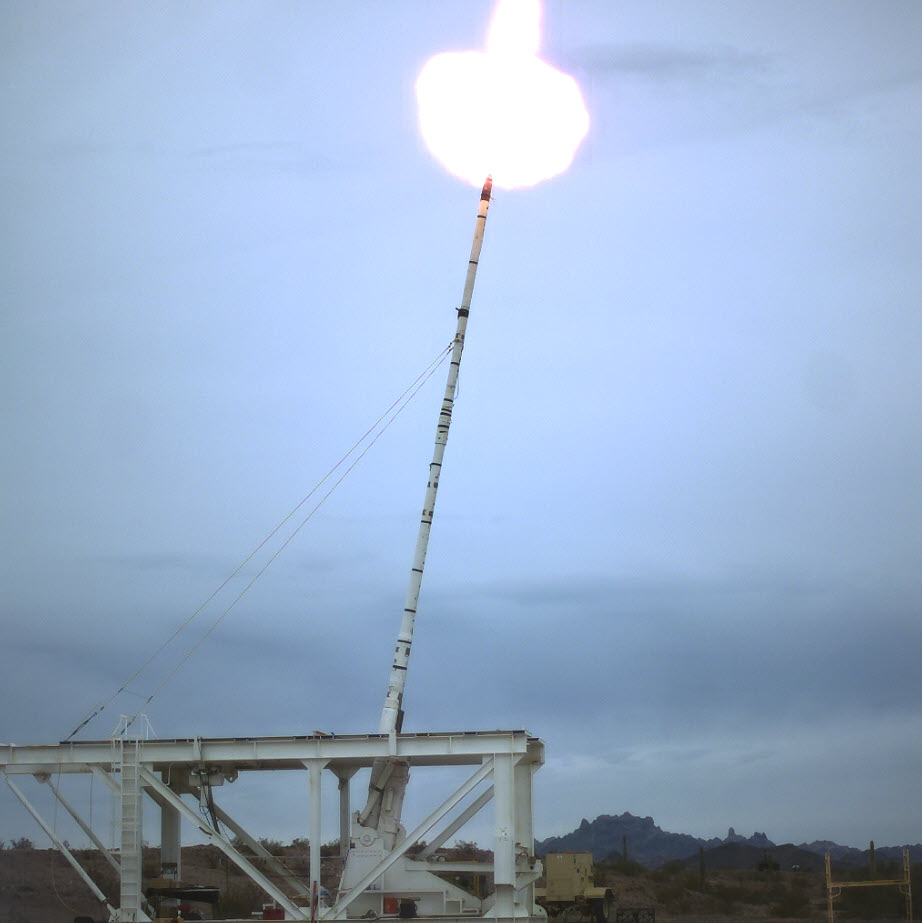
Problem #5: Gun-based ballistic launchers can achieve much greater exit speeds than SpinLaunch.
Although it’s a brilliant idea to try and cut out the first stage of a rocket, which after all is where the greatest fuel expenditures come from, SpinLaunch’s goals are impressive. With a launch speed of 5,000 mph (8,100 kph), it will certainly reach high altitudes on its own.
But why pioneer a technology that requires a large amount of expense, infrastructure, and moving parts — as well as requiring your payload to endure tens of thousands of gs for tens of minutes — when you can just scale up what we’ve already learned from Project HARP?
In the 1990s, Dr. John Hunter led what was known as Super HARP, which was a methane-and-hydrogen powered ballistic launch system that achieved exit velocities of 6,700 mph (10,800 kph). Rival startup Green Launch asserts that laboratory-based hydrogen systems have achieved exit velocities of 25,000 mph (39,600 kph) and that a full-scale projectile speed of 9,000 mph (14,400 kph) is accessible. In fact, a December 2021 test achieved exit velocities of 4,400 mph (7,200 kph): nearly matching SpinLaunch’s desired full-scale goals. While SpinLaunch will require at least two extra stages to reach space, Green Launch aims to reach the Kármán line that defines the start of space, 100 kilometers (62 miles) up, from the projectile launch alone.
There’s no doubt that there’s a lot of truth to the old saying that “fortune favors the bold,” and SpinLaunch is certainly a bold idea. However, the laws of physics pose numerous obstacles for those who would build high-powered, rapidly moving large-scale apparatuses with moving parts. In the 1990s, the Department of Energy attempted to build enormous centrifuges for accelerating large objects, but they always began to break down at speeds of ~3,100 mph (5,000 kph): about 60% of the speeds that SpinLaunch aims to achieve. The challenges before the team aiming to reach their stated goals are enormous.
That isn’t to say that SpinLaunch is impossible or that its concepts violate the laws of physics; they do not. However, there’s a very big distinction between what’s physically possible and what’s physically practical. It’s not clear that, with three times the diameter of the current prototype, the desired launch parameters can be met. Even if they are, it remains to be seen if the later-stages required to take the launched payloads to orbit can operate after experiencing the extreme SpinLaunch spin-up and launch and drag conditions. It’s important to explore a variety of options in the quest to reach space, but scaling up a prototype is rarely as easy as one might initially think.
This article was reprinted with permission of Big Think, where it was originally published.